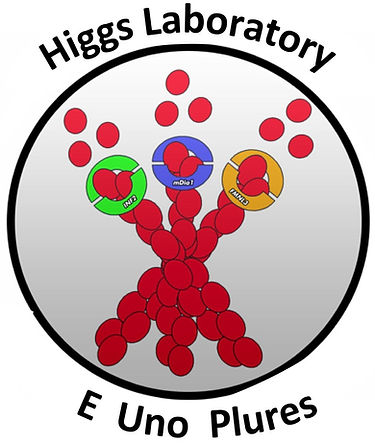
About Us
We strive to uncover novel connections in cell biology, starting from the basis of the actin cytoskeleton. Actin is an extremely abundant protein (about 200 micromolar in the cytosol of most mammalian cells) and can assemble into filaments extremely rapidly, almost anywhere. Some very prominent cellular structures are actin-based, such as stress fibers, lamellipodia and filopodia. The prevalence of these structures, however, somewhat overshadows the more subtle populations of filaments that are clearly serving important functions. Two examples of intense current interest in the lab are: endoplasmic reticulum-associated actin, and actin that polymerizes around mitochondria that are under stress. We are interested in the mechanisms that trigger this actin polymerization, and the functional consequences of this polymerization. The stress-induced actin is important metabolically, in that it activates glycolysis. We are trying to use this feature as an anti-cancer therapeutic.
​
Our lab motto is "from one, many":
a reflection on the number of roles
actin can perform in the cell.
​
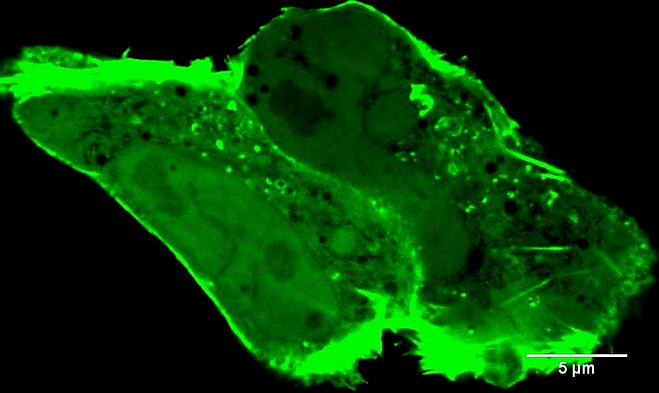
Endoplasmic reticulum-associated actin
​
Traditionally, the ER has not been thought of as a hotbed of actin. In the last few years, however, we and others have found that an ER-associated isoform of the formin INF2 can rapidly assemble actin filaments in response to a number of stimuli (see the video to the left). This “actin burst” has two immediate consequences that we know of: to stimulate transfer of calcium between ER and mitochondria (Chakrabarti et al (2018) J. Cell Biol); and to stimulate oligomerization of the dynamin GTPase Drp1, and its recruitment to mitochondria (Ji et al (2017) J. Cell Biol.). These events lead to mitochondrial division. Mitochondrial division is a key regulatory mechanism, controlling the activity these important cellular energy generators. In addition, the mitochondrial division machinery is important for two homeostatic processes: mitophagy and apoptosis. Defects in mitochondrial division are linked to multiple neurodegenerative diseases. Mutations in INF2 itself are linked to a neuropathy (Charcot-Marie-Tooth disease) and to a kidney disease (focal and segmental glomerulosclerosis). Our focus is to define three things in this process:
-
The activation mechanism of INF2. We now have exciting results showing that activation involves de-acetylation of actin! See Mu A et al, Nature Cell Biology (2019) and Mu A et al, PNAS (2020) for details. The regulatory mechanisms of actin acetylation/deacetylation on INF2 both biochemically and in cells are of very active interest to us.
-
The mechanisms by which INF2-mediated actin filaments stimulate mitochondrial division. We have recently found that actin synergizes with the Drp1 receptor Mff to stimulate Drp1 activation (Liu et al, Mol. Biol. Cell (2021)). Further studies will define the minimal set of components needed for full Drp1 activation and recruitment biochemically, and will determine whether actin synergizes with other Drp1 receptors (MiD49 and MiD51).
Actin polymerization around stressed mitochondria
In addition to INF2-mediated actin filaments, a very different population of actin has very different effects on mitochondria. When mitochondria are stressed, they often get "depolarized." In other words, they lose their membrane potential, due to loss of electron transport chain activity which provides the proton gradient across the inner mitochondrial membrane (IMM). A very early response to mitochondrial depolarization is assembly of a dense actin “cloud” around the mitochondrion, which we call “ADA” (Acute Damage-induced Actin). ADA is transient, fully polymerizing within 5 min and depolymerized by 10 min. We now know that a wide range of stimuli can induce ADA, including: mitochondrial depolarization (CCCP), electron transport chain inhibition (antimycin, rotenone), ATP synthase inhibition (oligomycin), the anti-diabetes drug metformin, and hypoxia (Fung et al Curr. Biol. (2022), Chakrabarti et al Biorxiv (2022)). We have shown that ADA is independent of INF2, but depends upon another actin polymerization factor, Arp2/3 complex (Fung et al J. Cell Sci. (2019)). At present, we have elucidated two major effects of ADA: 1) glycolytic activation, and 2) inhibition of mitochondrial dynamics. We are actively following up these findings.

In all of our research, we use a combination of cell-free biochemistry, live-cell microscopy, and other techniques such as fractionation of cellular extracts. We are trying to push our biochemical studies more toward reconstitution now, using full-length proteins under their proper regulation. These studies have lead to some striking insights that will be the subject of publications yet-to-come. Eventually, I hope, these will lead to cell-free reconstitution of ER-associated and mitochondrially-associated actin polymerization in the future.
Our live-cell studies are focused on establishing well-controlled, reproducible systems that can be quantified and used as reliable bio-assays to test biochemical predictions (“cellular biochemistry”). These experiments generate some striking images, but that is not our focus. In the era of the sound bites and tweets and “look at me!” science, we strive to take the long view. Of course, we’re not averse to the occasional tweet as well!
​
Learn more about the basics of actin biochemistry!
​
​
ADA in T cell function and cancer therapeutics
One very interesting facet of ADA is that it is important for glycolytic activation in CD8+ effector T lymphocytes (Teffs). These cells are quite dependent on glycolysis anyway, and are important for our own immunological response to cancer. Adoptive T cell therapies (such as CAR-T) have proven effective against a range of cancers, but are less effective in solid tumors, with one reason being that Teffs are out-competed for glucose by the cancer cells themselves. We are testing the effect of boosting ADA in Teffs ex vivo during adoptive T cell therapy, as a way of improving their efficacy. These are collaborative studies with Ed Usherwood at Dartmouth.
​
Images to the left are Teffs stimulated with the indicated treatments that induce ADA (AA = antimycin, Rot = rotenone) and stained for mitochondria, actin and DNA. Note actin around the mitochondria in the stimulated cells. Scale bar = 5 microns in full cell images and 2 microns in zooms. Teffs are small cells, tough to image!